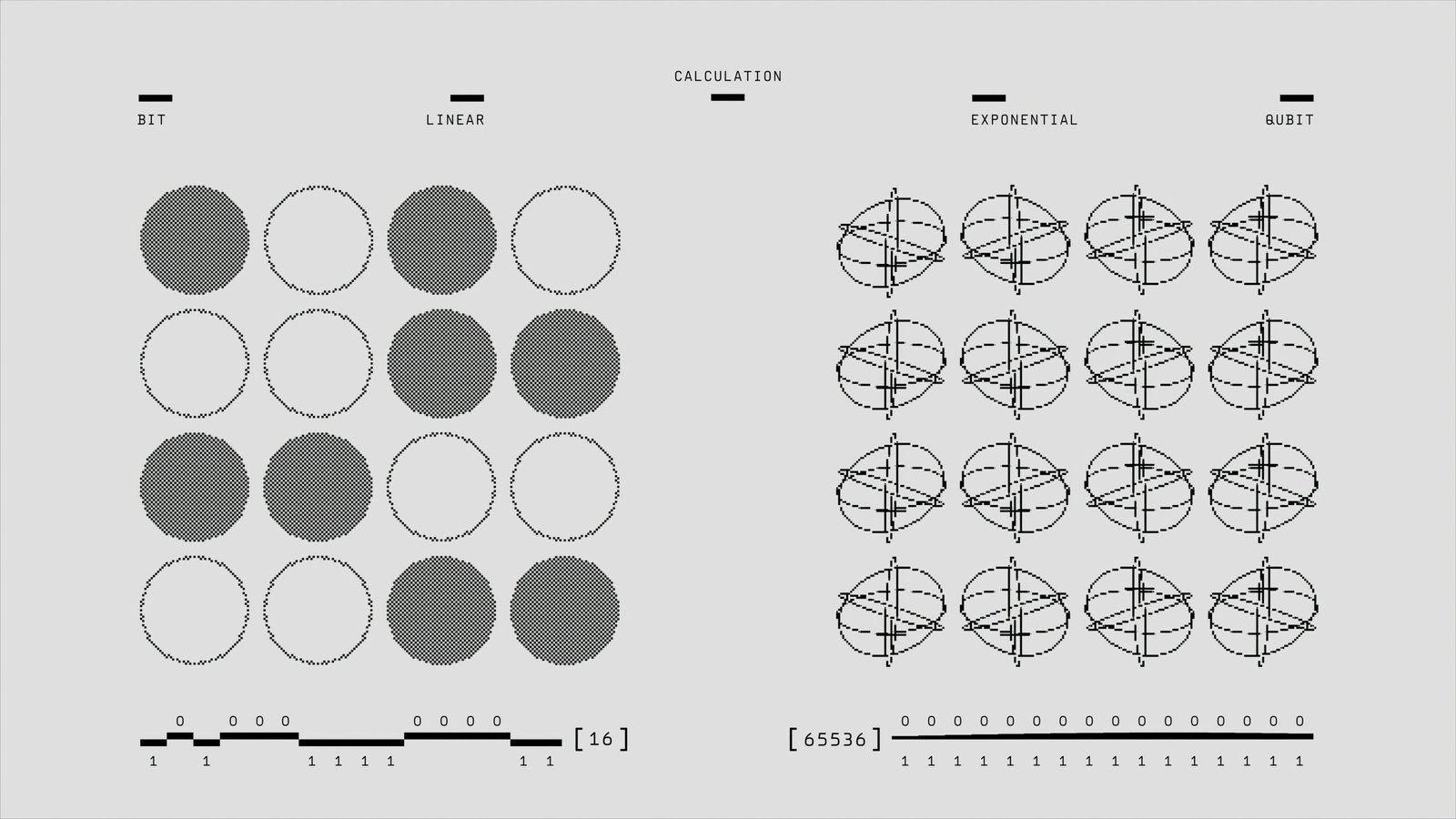
What is Quantum Computing?
Quantum computing represents a paradigm shift in how computations are performed, fundamentally distinguishing itself from classical computing. The essence of traditional computing relies on bits, which can exist in one of two discrete states – 0 or 1. In contrast, quantum computing utilizes quantum bits, known as qubits, which harness the principles of quantum mechanics. A qubit possesses the unique ability to exist in multiple states simultaneously, thanks to a property called superposition. This characteristic enables quantum computers to process information in ways that classical computers simply cannot.
To further understand the functionality of qubits, it is essential to recognize that they can represent both 0 and 1 at the same time. This superposition allows quantum computers to perform numerous calculations concurrently, vastly increasing their computational power for certain tasks. For example, while a classical computer would have to evaluate all potential solutions to a given problem sequentially, a quantum computer can manage multiple possibilities simultaneously, accelerating problem-solving significantly.
Moreover, quantum computing also involves another critical principle known as entanglement. When qubits become entangled, the state of one qubit becomes dependent on the state of another, regardless of the distance between them. This interdependence allows quantum computers to perform complex calculations that would be impractical for classical systems, further underscoring the transformative potential of quantum technologies.
The advancement of quantum computing heralds numerous applications across various fields, including cryptography, material science, and complex system modeling. As researchers continue to explore the possibilities of quantum computing, it becomes increasingly clear that this technology has the potential to revolutionize the landscape of problem-solving and data analysis, opening new frontiers inaccessible to classical computing methodologies.
The Historical Context of Quantum Computing
The evolution of computing can be traced back to the early 19th century, when Charles Babbage designed the Analytical Engine, often regarded as the first mechanical computer. This paved the way for further innovations in computation, culminating in the development of electronic computers during the mid-20th century. The seminal work of Alan Turing, often referred to as the father of computer science, offered a theoretical foundation that dictated modern computing principles, primarily through the conception of the Turing machine.
As the field matured, notable figures, such as John von Neumann and Claude Shannon, significantly contributed to the architecture and information theory that underpin today’s computers. However, a new paradigm in computing began to emerge in the latter half of the 20th century, as physicists started to explore the principles of quantum mechanics. The theoretical groundwork that led to quantum computing was laid by individuals such as Richard Feynman and David Deutsch, who recognized that classical computers struggled to simulate quantum mechanical systems efficiently. In 1981, Feynman proposed that quantum systems could be harnessed to perform computations in a fundamentally different way, leading to the exploration of quantum algorithms.
By the 1990s, the practical implications of quantum computing gained traction, particularly with the development of Shor’s algorithm, which demonstrated that quantum computers could factor large numbers exponentially faster than their classical counterparts. This breakthrough not only showcased the potential of quantum technology but also raised questions about the implications for encryption and security in the digital age.
As we look towards the future, it is clear that the progression from classical computing to quantum computing is rooted in a rich historical context, grounded in the interplay between theoretical physics and practical computation. The milestones achieved thus far provide a compelling narrative that showcases humanity’s quest to understand and leverage the laws of quantum mechanics for transformative computational capabilities.
Key Principles of Quantum Mechanics
Quantum computing is intrinsically linked to the principles of quantum mechanics, which govern the behavior of matter and energy at extremely small scales. Understanding these principles is essential for grasping how quantum computers operate and their potential to surpass traditional computing capabilities. Three fundamental concepts that underpin quantum mechanics are quantum superposition, entanglement, and quantum interference. Each of these concepts plays a pivotal role in enabling the unique functioning of quantum computers.
Firstly, quantum superposition refers to the ability of quantum bits, or qubits, to exist in multiple states simultaneously. Unlike classical bits that can either be 0 or 1, qubits can be in a state where they represent both 0 and 1 at the same time. This multiplicity of states allows quantum computers to perform multiple calculations concurrently, thereby accelerating problem-solving processes. Superposition is crucial for the development of quantum algorithms that can efficiently tackle complex problems.
Secondly, entanglement is a phenomenon where pairs of qubits become interconnected in such a way that the state of one qubit instantly influences the state of another, regardless of the distance between them. This peculiar relationship enables quantum computers to perform operations that are exponentially more efficient than those possible with classical computers. The power of entanglement is harnessed in various quantum algorithms, allowing for more streamlined data processing.
Lastly, quantum interference is essential in determining the probabilities of various outcomes within quantum algorithms. When applied appropriately, interference can amplify the probabilities of correct answers while minimizing the likelihood of incorrect ones. This mechanism is crucial for the success of quantum computing, as it contributes to the accuracy and reliability of computations.
These key principles of quantum mechanics form the backbone of quantum computing, offering insights into how this revolutionary technology operates and its potential applications across various fields.
How Quantum Computers Work
Quantum computers operate on principles fundamentally different from classical computers. While classical bits represent information as either a 0 or a 1, quantum computing utilizes qubits, which can exist simultaneously in multiple states due to the phenomenon known as superposition. This unique characteristic enables quantum systems to perform complex calculations at exponentially faster rates than traditional machines.
The architecture of quantum computers is designed to harness the power of qubits effectively. A typical quantum system includes a quantum processor composed of numerous qubits, which are often realized using superconducting circuits, trapped ions, or photons. These qubits are manipulated using quantum gates, which function like classical logic gates but allow for the simultaneous processing of multiple combinations of states. Quantum gates can entangle qubits, creating a correlation between them that is critical for the performance of quantum algorithms.
When a quantum computation is carried out, the manipulation of qubits through quantum gates produces a quantum state that encodes the solution to a problem. Subsequently, the quantum measurement process collapses the superposed states into a definitive classical state, allowing for the reading of the calculated result. This measurement is fundamentally probabilistic, meaning that multiple runs might be necessary to achieve a high confidence level of a given outcome.
Numerous quantum algorithms have been developed to exploit these capabilities, including Shor’s algorithm for factoring large numbers and Grover’s algorithm for searching unsorted databases. These algorithms demonstrate that quantum computing has significant potential for solving specific problems much more efficiently than classical approaches. Overall, the interaction between qubits, quantum gates, and measurement processes forms the backbone of quantum computing technology, paving the way for innovations that could revolutionize numerous fields.
Current Applications of Quantum Computing
Quantum computing is at the forefront of technology, presenting innovative solutions across various fields. In cryptography, quantum computing holds the promise of enhancing security measures substantially. Quantum key distribution (QKD), which leverages the principles of quantum mechanics, allows users to share encryption keys securely, making eavesdropping virtually impossible. This application is considered a significant leap towards ensuring secure communication in an increasingly digital world.
Moving beyond cryptography, material science is another domain where quantum computing is making significant contributions. Researchers utilize quantum simulations to uncover the properties of new materials at an atomic level, leading to the discovery of superconductors and advanced nanomaterials. This could facilitate breakthroughs in the development of energy-efficient technologies and enhanced electronics, profoundly impacting how we understand and manipulate physical substances.
In the pharmaceutical industry, quantum computing presents intriguing opportunities for drug discovery and development. Traditional methods of simulating molecular interactions can be computationally intensive and time-consuming. However, quantum computing can process vast quantities of data and perform complex calculations at unprecedented speeds. This capability enables scientists to model biological reactions and identify potential drug candidates much faster, potentially speeding up the delivery of innovative treatments to the market.
Furthermore, optimization problems present another promising area where quantum computing can shine. From logistics and supply chain management to financial modeling, many sectors face complex optimization challenges. Quantum algorithms, such as the quantum approximate optimization algorithm (QAOA), provide more efficient solutions to these problems, significantly reducing the time and resources needed for decision-making processes.
As we continue to explore the potential of quantum computing, it becomes increasingly apparent that its applications could transform various industries, reshaping our technological landscape and enhancing our capabilities in tackling complex challenges.
Challenges and Limitations
Quantum computing, heralded as the next frontier in computational technology, faces a myriad of challenges that hinder its widespread implementation and advancement. One of the most significant of these is qubit coherence, which refers to the ability of a quantum system to maintain its quantum state over time. Coherence times are currently limited, and when a qubit loses its state due to environmental interference, it can lead to errors in calculations, thus jeopardizing the reliability of quantum computations. This issue demands robust error correction strategies that are still in the developmental phase.
Error rates present another substantial obstacle in the evolution of quantum computing. Quantum systems are inherently prone to noise and other disturbances that can induce decoherence, resulting in calculated results that may diverge significantly from intended outcomes. Addressing these error rates is crucial for the practical application of quantum computers; thus, ongoing research is dedicated to enhancing the precision of qubit operations and achieving fault tolerance.
The scalability of quantum systems poses yet another complex challenge. As the number of required qubits increases, so do the difficulties associated with maintaining stability and coherence across larger networks. Current quantum computers have been able to incorporate only a limited number of qubits, thereby restricting the complexity of problems they can effectively solve. The progression towards more extensive and capable quantum architectures is essential, yet it involves intricate engineering and technological advancements.
Furthermore, the quest for suitable quantum algorithms that can outpace their classical counterparts remains ongoing. While some quantum algorithms have shown promise, an understanding of their limitations when applied to practical problems is necessary. The exploration of new quantum algorithms and applications is critical to showcasing the advantages of quantum computing and justifying the resources invested in its development.
The Future of Quantum Computing
The landscape of quantum computing is steadily evolving, with numerous predictions surrounding its potential trajectory in the coming years. As researchers worldwide continue to explore this transformative technology, the anticipated breakthroughs in quantum algorithms and hardware are expected to facilitate more robust applications across various industries. These advancements could lead to solving complex problems that are currently infeasible for classic computational systems. A significant area of focus is the development of quantum supremacy, where quantum devices outperform classical computers in solving specific tasks.
Moreover, collaborative efforts among academic institutions, private enterprises, and governments are driving the advancement of quantum technologies. Initiatives such as public-private partnerships are paving the way for increased funding and resource sharing, thereby fostering innovative approaches to quantum computing research. This collaborative landscape is crucial for accelerating the maturation of quantum systems and ensuring that the lessons learned from current prototypes inform the next generation of quantum computers.
As quantum computing continues to mature, its societal implications warrant careful consideration. The introduction of quantum algorithms capable of breaking traditional cryptographic schemes raises critical questions regarding data security. Industries reliant on information protection, such as finance and healthcare, must adapt to these challenges through advanced cryptographic techniques that leverage quantum principles. Additionally, as quantum technologies become more accessible, there is potential for significant disruptions in sectors such as logistics, pharmaceuticals, and artificial intelligence.
Overall, the future of quantum computing is brimming with possibilities. While obstacles remain, the collective effort of the global research community is likely to unlock new frontiers in technology that we have only begun to imagine. This transformative evolution may not only redefine computational capabilities but also alter the framework of various industries and everyday life.
Getting Started with Quantum Computing
For individuals interested in embarking on the journey into the world of quantum computing, the first step is to familiarize themselves with the fundamental concepts. A good starting point is to explore online courses that cater specifically to beginners. Platforms such as Coursera, edX, and Udacity offer structured courses designed by leading institutions, enabling learners to grasp both the theoretical and practical aspects of quantum mechanics and its applications in computation.
In addition to online courses, there are numerous books available that provide an introduction to quantum computing. Titles such as “Quantum Computation and Quantum Information” by Michael Nielsen and Isaac Chuang serve as quintessential texts in the field, presenting complex ideas in a digestible format. Other recommended readings also include “Quantum Computing for Computer Scientists” by Noson S. Yanofsky and Mirco A. Mannucci, which connects theoretical foundations to computational practices in a clear, accessible manner.
Another valuable resource for beginners is to explore software development kits (SDKs) tailored for quantum programming. IBM Q Experience, for example, grants users access to real quantum computers and allows them to run experiments and develop quantum algorithms through its user-friendly interface. Additionally, Qiskit, an open-source quantum computing framework from IBM, provides tools for creating simple programs and running simulations, making it ideal for novice learners eager to practice their skills.
Participating in community forums can also be beneficial as they offer a platform for novice quantum enthusiasts to connect, share knowledge, and seek assistance. Engaging on platforms like Stack Overflow, Reddit, or the Quantum Computing Stack Exchange can enhance learning and enable individuals to stay updated on the latest developments in the field.
By leveraging these resources, beginners can build a solid foundation in quantum computing, opening the door to a future of endless possibilities in this fascinating and rapidly evolving domain.
Conclusion
As we have explored throughout this blog post, quantum computing represents a significant leap forward in computational capabilities compared to classical computing. By harnessing the unique properties of quantum bits, or qubits, this advanced technology offers unprecedented processing power, which is essential for solving complex problems that are currently beyond our reach.
The discussion has highlighted the fundamental principles of quantum mechanics that lay the groundwork for quantum computing, such as superposition and entanglement. Understanding these concepts is crucial for grasping the potential applications of quantum computing across various fields, including cryptography, drug discovery, and optimization challenges. As industries increasingly recognize the profound implications of quantum technologies, staying informed about developments in this area becomes increasingly important.
In a technology-driven world, engaging with quantum computing is not just for specialists in the field; it is an opportunity for anyone interested in the frontier of technology. Scholars, professionals, and enthusiasts alike are encouraged to seek out educational resources, participate in discussions, and consider contributing to research initiatives. The evolution of quantum computing is poised to disrupt numerous sectors, and understanding its fundamentals will be invaluable as we approach this new era. By fostering a culture of inquiry and learning about quantum technologies, we can collectively navigate the challenges and opportunities this innovative field presents.